Motor Proteins - Sophisticated Nanomotors in Our Body
“Assoc. Prof. Mert Gur of the Mechanical Engineering Department is studying nano scaled biological engines, known as motor proteins, that use fuel (ATP molecule) to generate mechanical movement to transport intracellular cargos. By understanding their mechanism of action at the atomic level, he aims to provide crucial information about how these motors carry out their cellular roles and to open up new avenues for the use of these motors in nanotechnology applications."
All of the important destinations within our cells are connected through tiny tracks called microtubules. Biological nanomotors, called motor proteins, transport cargo along these tracks, similar to trucks transporting cargos along highways, to ensure that cells are functioning properly. As it is on highways, cargo transport along microtubules is performed in two directions; towards the minus and plus ends of the microtubules. Dyneins perform most of the minus-end cargo transport in cells, while kinesin and myosin perform plus-end transport. Loss of dynein function has been associated with numerous neurodegenerative diseases and disorders, including ALS, Alzheimer's disease, lissencephaly, and schizophrenia.
Dyneins are nano scaled cellular engines that function similar to car engines. They take fuel (ATP molecule) as input, extracts its chemical energy via a chemical reaction (ATP hydrolysis), and transform the extracted energy via its complex machinery into mechanical energy in the form of the motion of a mechanical component (called linker) to generate force and motion. The linker moves forth and back between two positions and produces power, which is similar to the piston head motion and power production of a car engine. The produced mechanical energy can then be used to transport cargo, prepare the cell for mitosis, or move cilia. As it is for a car engine, dyneins operate on thermodynamic cycles; they start at one state, perform their function, return to the same state to repeat their cycle. As a car driver can control the revolutions per minute of a car engine (i.e. its thermodynamics cycles per minute), dynein’s thermodynamic cycle per unit time can be controlled via various external parameters by the cell, such as ATP concentration, microtubule associated proteins and regulatory factors. Since synthetic nanomotors that can covert the chemical energy into the motion of an internal mechanical component do not exist, dyneins are expected to play a critical role in the future of nanotechnology.
Gur has been leading one The Scientific and Technological Research Council of Turkey (TUBITAK), two Partnership for Advanced Computing in Europe (PRACE), and one Istanbul Technical University BAP projects performed in collaboration with UC Berkeley (USA) and Medical Research Council (UK), with the aim of modelling dynein’s thermodynamic cycle. Their first collaborative work was published in 2019 in Nature, where they explored how dynein’s minus end directionality is controlled and engineered dyneins which are able to walk toward the plus end of the microtubule.
To understand exactly how dyneins work, Gur uses molecular dynamics simulations to fill in the gaps in experimental methods. Although experiments are able to provide critical knowledge regarding protein functions, they are not able to visualize the dynamics at the atomic resolution. For example, electron microscopy can provide near-atomic structure of proteins, by trapping the protein at one state along the thermodynamic cycle. Even if multiple structures along the pathway could be obtained, it is hard to connect atomic structures to obtain the full dynamic picture of the thermodynamic cycle. This is similar to taking multiple pictures of a person on a bicycle along various locations. Based on a few pictures, it could be theoretically claimed that the bicycle might be jumping or sliding to go from one location to the other. However, in reality the person on the bike is moving her/his legs and turning the tires through a mechanism. In collaboration with his experimental collaborators, Gur and his research group are performing Molecular dynamics simulations to explore the atomistic details of protein functions.
Cytoplasmic dynein is composed of two identical dynein heavy chains (DHCs), which are connected to each other by their linkers through a common tail, and also several smaller associated polypeptides. Dyneins move along microtubules with a stepping mechanism where one DHC takes a step while the other DHC holds on to the microtubule. The DHC bound to the microtubule undergoes a thermodynamic cycle and performs the mechanical work required to pull the cargo; called the dynein power stroke. After the power stroke, DHC releases from the microtubule to complete its thermodynamic cycle (priming stroke) and advances towards the transport direction on the microtubule.
So far, Gur and his colleagues have successfully modelled the priming stroke of the linker, showing in detail the mechanism and energetics of this process. They have also modelled how dynein interacts with specific residues on tubulin and stably binds to microtubules. Gur and his collaborators have studied how microtubule-associated proteins (MAPs) regulate microtubule binding of dyneins and also kinesin. His recent study in collaboration with UC Berkeley, University of Washington, Howard Hughes Medical Institute, and TU Delft showing the mechanism of kinesin regulation by MAP7 has been accepted for publication in Science.
The system of the dynein molecule solvated in water is at least ~one million atoms in size and goes up to multimillions atoms if microtubule and associated proteins are included. This is least 10-100 orders larger than generally performed standard molecular dynamics simulated protein systems. Therefore, only a limited number of research groups have the massive resources to work on these systems, which are currently provided to Gur by PRACE. He has performed some of the first molecular dynamics simulations of dyneins and performed a significant contribution to the field since dynein’s mechanochemical cycle has not been simulated at atomistic detail before.
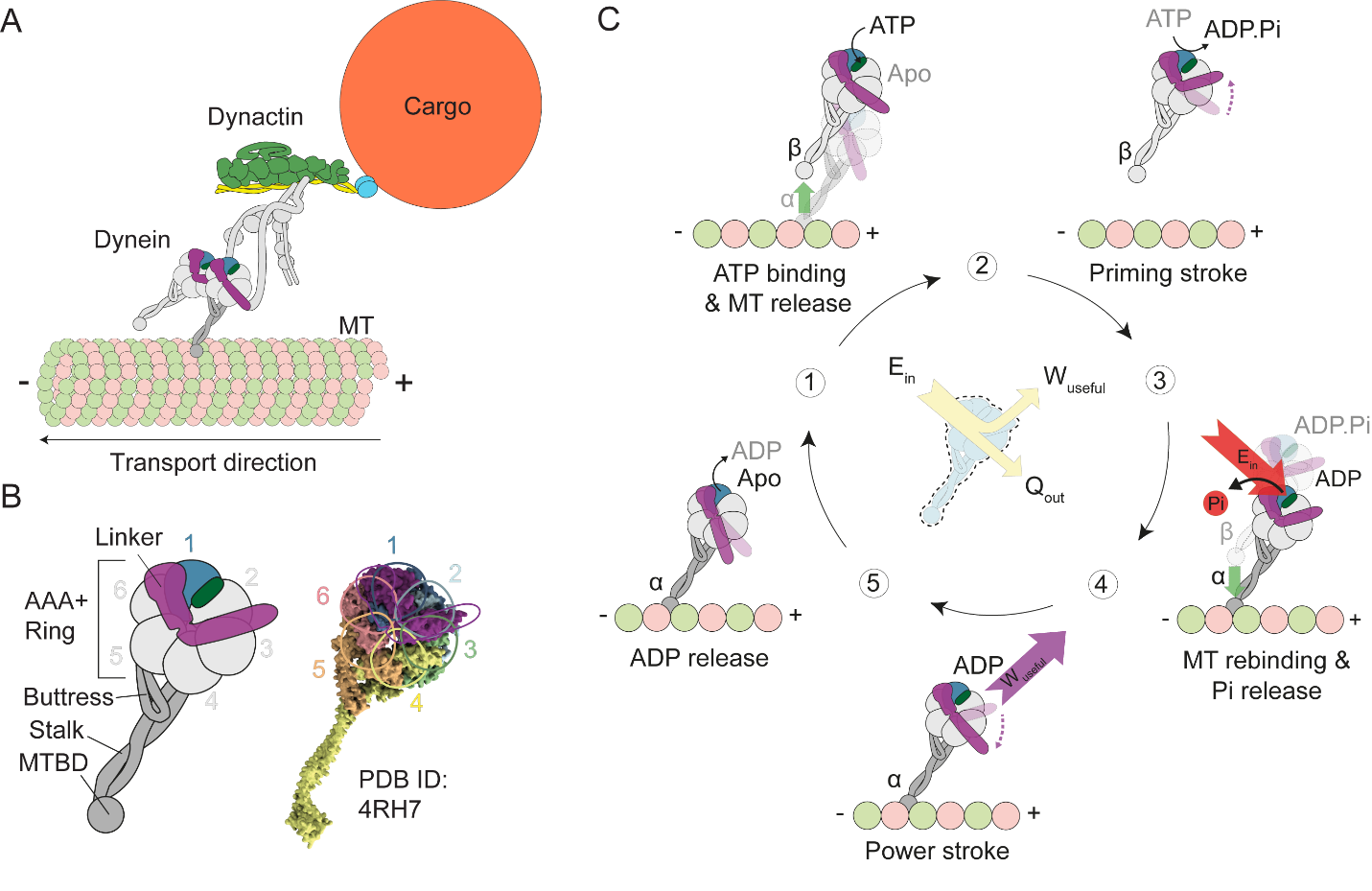
Figure 1. Dynein structure and mechanochemical cycle. A) Dynein pulling cargo via stepping along the MT. B) Structure and domain separation of each DHC. C) The mechanochemical cycle of the DHC. Linker undergoes ATP dependent conformational changes and generates force and motility, synchronized with MT binding/unbinding cycles.
Video 1. Engineering plus end directed dynein constructs. Related Articles:
1. Can, S., Lacey, S., Gur, M., Carter, A. P., Yildiz, A. (2019). Directionality of dynein is controlled by the angle and length of its stalk. Nature, 566(7744), 407.
2. Ferro, L., Eshun-Wilson, L., Golcuk, M., Fernandes, J., Huijben, T., Gerber, E., Jack, A., Costa, K., Gur, M., Fang, Q., Nogales, E. and Yildiz, A. (2020). The mechanism of motor inhibition by microtubule-associated proteins. bioRxiv. (Preprint).
3. Ferro, L.S, Fang Q., Eshun-Wilson, L., Fernandes, J., Jack, A., Farrell, D.P., Golcuk, M., Huijben, T., Costa, K., Gur, M., DiMaio, F., Nogales, E., Yildiz, A. (2022). The mechanism of biphasic regulation of kinesin-1 by MAP7. Science. (Accepted)
Fundings:
Tubitak (3501, Grant No: 215Z398; 2247,121C283; 2501, 122N045), ITU BAP (Grant No: MGA-2021-42803), and PRACE DECI-17 (Grant No:17DECI0080), PRACE (Grant No: 2019215144, 2021250119),
Collabrators:
Prof. Ahmet Yildiz (UC Berkeley, USA), Prof. Eva Nogales (UC Berkeley, USA), Dr. Andrew P. Carter (MRC Laboratory, UK), and Dr. Simon Bullock (MRC Laboratory, UK)
Lab Members:
Mert Golcuk, Sema Zeynep Yilmaz, Reyhan Metin Akkaya, Clara Xazal Buran, Derman Basturk, Cihan Ugur Otcu, Ayla Eren, Ebru Tuncay